Key insights from
Livewired: The Inside Story of the
Ever-Changing Brain
By
David Eagleman
|
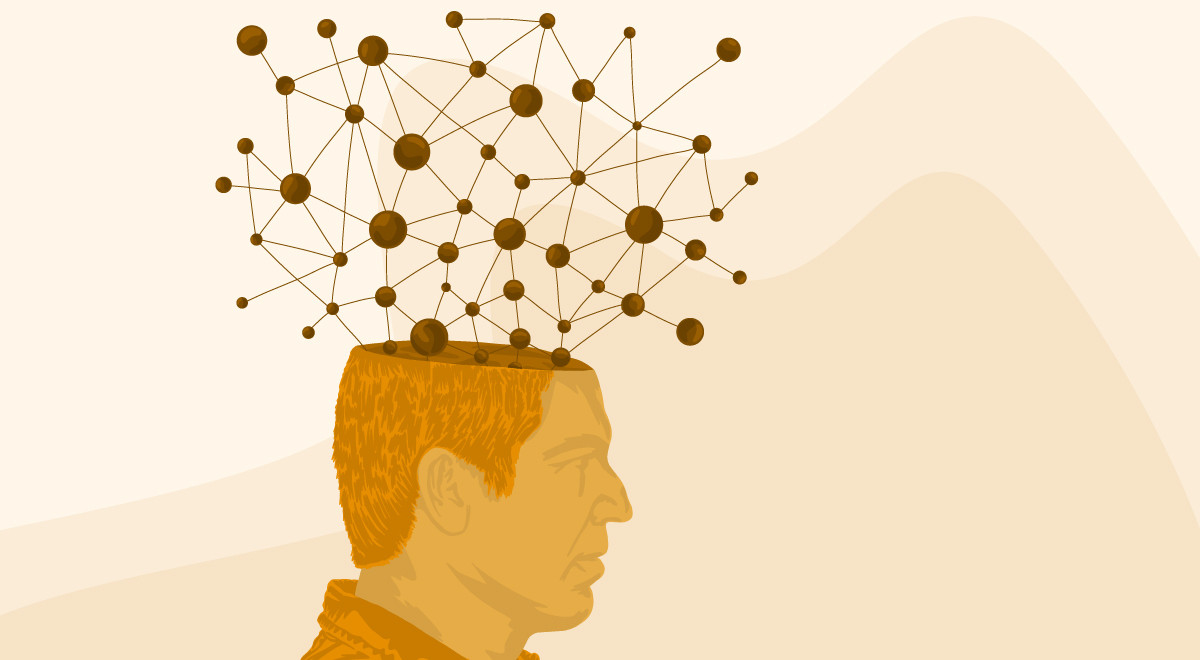
|
|
What you’ll learn
86 billion neurons weave
constellations of meaning. Our brains are much more than this mass
accumulation of neuronal activity, though—rather, they’re breathing,
evolving systems that learn to create sensory reality from mere
information. The same organ that enables you to pick up subliminal social
cues on a date allows amputees to operate robotic arms with relative ease.
Neuroscientists are only just beginning to explore these remarkable
capabilities of the constantly shifting human brain. Renowned writer and
neuroscientist David Eagleman brings readers to the cusp of new research in
the realm of brain plasticity, connecting the dots of our neural portraits
and sparking an internal realization: We are much more than we think.
Read
on for key insights from Livewired: The Inside Story of the Ever-Changing
Brain.
|
|
Sponsored
by Revtown
Ex-Under Armour
Execs are making jeans.
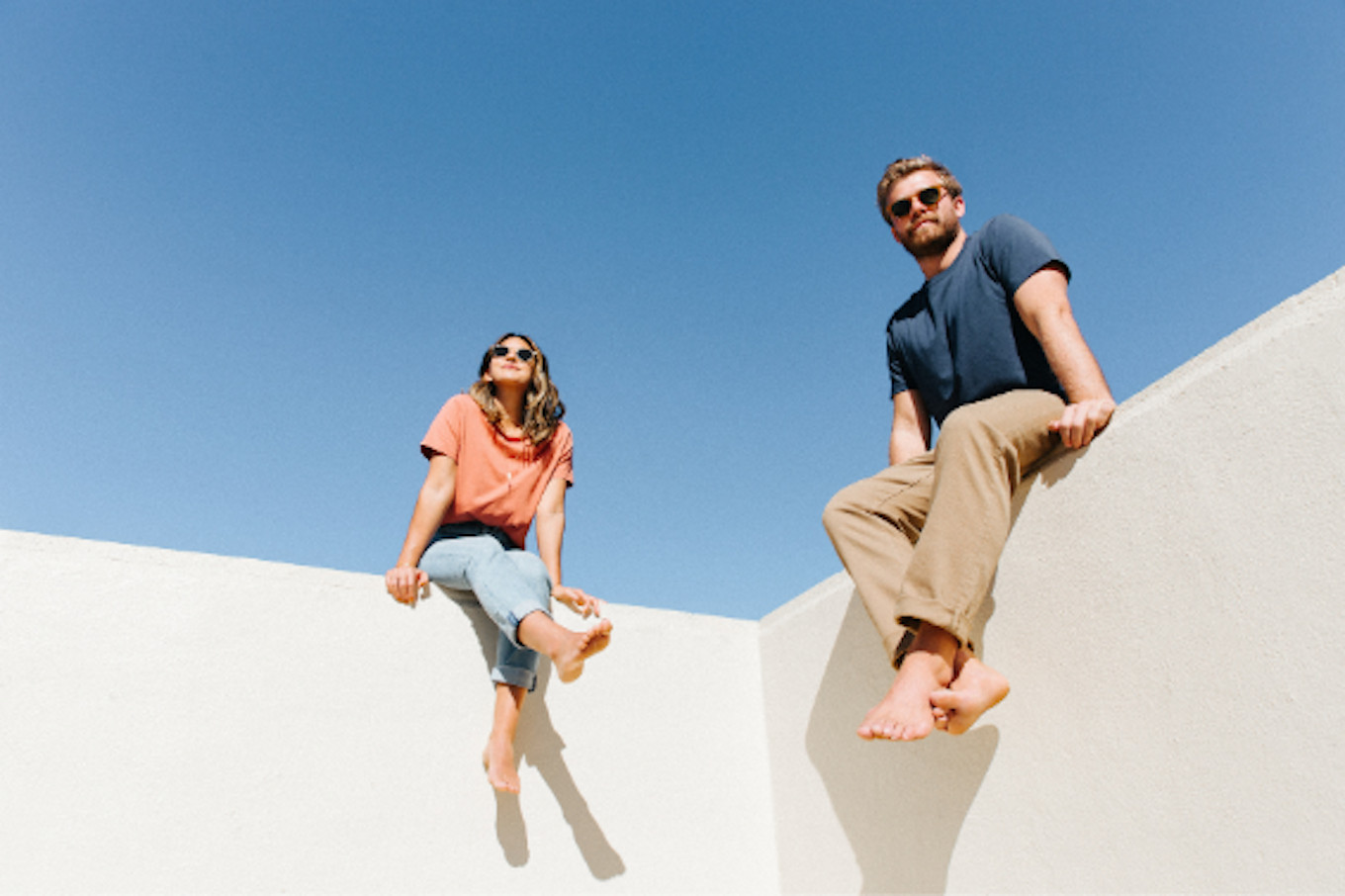
How? by using a proprietary fabric
consisting of Italian milled denim infused with the same stuff used in
football uniforms and yoga pants. Revtown jeans are a triple threat,
sustainable, comfortable, and affordable.
Shop Good Jeans
|
|
1. New day, new
you—literally.
What were you doing this
time last year? Who were you with? What were you thinking about? Your
answers might reflect those of a person entirely different from who you are
now. According to the author, each of us is a product of a constantly
shifting brain. William James, a famed psychologist of the late 19th
century, called this aspect of the evolving brain its “plasticity.” Though
brain plasticity is an accurate and proven scientific fact, the author
prefers a different descriptor that’s better suited to the way the brain
behaves within its environment: Our brains are “livewired.” The brain isn’t
playdough, a pink putty that holds shape after we mold it to our liking;
rather, the brain is a house under constant reconstruction, eluding our
awareness with its perpetually morphing form.
The brain’s highly flexible
and incomplete shape makes it amenable to adapt to whatever environment it
finds itself within. Infants don’t stumble into this world with a brain
that can already make sense of its surroundings, and know the meaning of
its mother’s smile or the solutions to differential equations. The brain
must learn about and use the world in order to survive. Our 20,000 genes
provide our 200 trillion networking neurons a canvas upon which to paint a
world and an experience. This is what makes the formative years, also
called the sensitive period, of infancy and early childhood so potent—the
environment of your 2-year-old self influences the wellness of your
52-year-old self.
Cases in which the nurture
part of the “nature vs. nurture” argument were neglected are harrowing. In
2005, police in Florida uncovered one such instance of child abuse after
receiving calls from neighbors who had spotted a small girl they’d never
seen before in a window of a nearby home. The girl’s name is Danielle
Crockett. She was just a tiny, nearly 7-year-old child at the time she was
found, covered in her own excrement and bugs, locked in a closet for her
entire life. Danielle failed to receive adequate nutrition, love, and input
during those early years, so despite her rescue, it was unfortunately too
late for her brain.
Danielle, a feral child,
lives with no discernible mental disabilities but still can’t use the
restroom on her own or complete seemingly simple tasks. Her brain failed to
receive the necessary input during a highly crucial stage of her
development. Both the external world and interactions with people are
integral to the brain’s growth, and this requirement is most critical in
childhood when the brain is just forming to know itself, its environment,
and the body it will learn to lead.
|
|
2. It’s a
beautiful day in the neuronal neighborhood: The organization of the brain
replicates that of the body.
The layout of our bodies
influences the outline of our brains. The neurosurgeon Wilder Penfield
uncovered this hardly realistic aspect of the brain in 1951, calling his
blueprint the “homunculus” which means “little man.” Penfield found that
the section of the brain in charge of receiving inputs—the somatosensory
cortex—and the part of the brain in charge of dictating outputs—the motor
cortex—both embodied the human body. For instance, sensations felt along
the spaces of one’s leg to one’s toes were stimulated by similarly close
areas in the brain. Body parts that are near each other in human anatomy
trigger places in the brain that parallel their closeness.
Later (ethically dubious)
experiments on Silver Spring monkeys headed by neuroscientist Edward Taub
in the 1990s proved that these little mental maps weren’t formed at birth.
Rather, just like the brain itself, the homunculus modifies itself based on
bodily inputs. The author notes that as neurons fire, they send electrical
pulses called spikes. These spikes network with 10,000 other neurons. When
one neuron’s spike is followed by another neuron’s spike, a bond is forged.
Soon, much like a newly-married couple, the neurons settle down together,
inhabiting a similar area of the brain, and deepening their relationship
through the mutual giving and receiving of neurotransmitters.
Just like our homunculus
replicates the spacing of our typically standard bodies due to neuronal
spikes, our homunculus also changes to reflect a less conventional body.
Our neurons rush to rearrange our cranial rooms at the slightest hint of a
change, to best reflect our environment and suit our senses to derive
information from such. Researcher of neurology Alvaro Pascual-Leone
conducted a study to chart the speed with which the brain responded to
these changing inputs, blindfolding participants for 5 days in order to
simulate blindness. These blindfolded people were then trained in Braille
alongside a control group of people who were not blindfolded.
It took only 5 days for the
brain to respond in crucial ways. The researchers studied the brains of the
blindfolded participants and saw that their occipital cortex, the section
of the brain involved in sight, was actually repurposed when they touched
the Braille letters. Meanwhile, those without blindfolds employed their
somatosensory cortex only and were less successful at reading Braille. Due
to the participants’ temporary loss of sight, the brain rewove and redeemed
itself to make sense of the stimuli it received—loss sharpened what
remained.
This is why blind musicians
are more likely to have perfect pitch than musicians with normal sight, and
why in one study, they even scored higher on a memory test. Their occipital
cortex is used to enhance the senses they still have. Nothing lost is ever
really lost—not to the human brain at least.
|
|
3. We dream to
avoid death—maybe.
Dreams are realms of
ambiguity—being chased by monsters, failing an exam, showing up to a party
without clothes—science has little understanding of why we conjure these
vivid depictions during the dark hours of the night. The author may have an
answer, and it brings us back to the functioning of our occipital cortex.
When a body part isn’t used or receives no stimuli, its space within the
brain atrophies and rearranges itself to support incoming information.
Here’s where dreaming might save us from dying.
A majority of human history
lived in an average of twelve hours of darkness per planetary cycle. Now we
have lamps, lights, and modern electricity, but before, our brains had to
fight the destruction of the occipital lobe every night. The author postulates
that the brain avoided the loss of its visual function through contriving
those elaborate, colorful dreams of REM sleep. Neurons in the part of the
brainstem called the pons activate REM sleep, locking the body into
paralysis and shooting neuron spikes to the occipital cortex. Think of it
as a personal, internal movie theater—one where you have no clue what film
might play and no ability to stand up and walk out if it’s a bust.
To preserve one’s ability
to see, the brain stages these nightly movies of electrical circuitry to
the lateral geniculate nucleus. The action doesn’t happen anywhere else,
either—a factor the author views as strong evidence of its particular and
integral function. Similarly, the experience of REM sleep in other
organisms, both “immature” and “mature” species, solidifies this fact.
“Immature” organisms like humans and ferrets are birthed into an unknown
world and must learn and adapt to their environments in order to survive.
“Mature” animals like giraffes arrive ready to handle what their
environment may throw at them. Immature animals need about 8 times more REM
sleep due to what the author argues is the formability of the early brain,
which must work to maintain itself. This might also be why babies spend
half of their rest in REM sleep, while adults only require 10-20% of REM.
Less moldability—less dreaming.
Still, we all dream to a
certain extent—our brains haven’t shed the restful necessity of REM. This is
true even for those who are born blind. Instead of visual information
displayed within their occipital cortex, they receive other dreamlike
experiences, such as that of walking through a room. Dreams may seem like
odd conglomerations of your worst fears, forgotten memories, or distant
hopes, but these electric medleys may serve a purpose—even if you can’t
remember them.
|
|
4. Sensory
extensions aren’t the stuff of fantasy; the brain can do much more than we
think.
What does the color red
sound like? Or how might electromagnetic waves feel on the tips of your
fingers? The answers to those questions might always be a mystery, but that
doesn’t mean the brain couldn’t figure them out if given the proper
equipment. The experience of a painting or the sun on our shoulders
consists of electrochemical pulses that arrive at various organs that relay
information to the brain. Our experiences are dictated by our genetics,
which comprise the layout of our bodily senses. The author’s Potato Head
hypothesis for this phenomenon states that it doesn’t matter what organ
transmits the signal to the brain; the brain will always employ bodily
organs to create meaning out of mere electrochemical sparks.
The neuroscientist Paul
Bach-y-Rita hailed what the author terms the advent of “sensory
substitution,” with his groundbreaking research. Bach-y-Rita’s experiments
concluded that the brain could employ input arriving from the skin to
produce something similar to sight. Contemporary developments in sensory
substitution operate within the same principle: The brain’s cortex is
“pluripotent,” which means that its use can manifest in many ways depending
on the available resources. The contemporary Neosensory Buzz wristband
employs electrochemical pulses to replicate sound through vibrations for
deaf people, translating their environments and bringing them to life.
Besides this widely useful
method of sensory substitution, experiments in human sensory experience
also have given way to “sensory enhancement” and “sensory addition.” Both
methods use the relationship between the formable brain and senses to
provide more vivid or wholly novel experiences of the world. Tapping into
the pliable brain unleashes a world of seemingly fictional human abilities.
The biohacker Todd Huffman placed a tiny neodymium magnet beneath his skin
in order to sense the magnetic waves that constantly surround us but fly
below the level of our sensory perception. Huffman says that his new
ability feels as if he’s tapping a bubble—a slightly frustrating occurrence
when you’re trying to fry an egg on your stove.
These seemingly utopian
fantasies prove that the brain is a scavenger—it takes whatever it can get
to manifest an experience. Our bodies aren’t able to soak in all the
electrochemical signals that stitch our lives together, but if they could,
our brains would surely use them.
|
|
5. Arguing neurons
bring bodily peace.
At three years old, Alice
seemed like an ordinary little girl, but after she started experiencing
seizures, her parents sought medical help. The results of a brain scan
showed a terrifying realization, one that would shake even the bravest of
parents: Alice was missing half her brain. Her entire right hemisphere was
completely gone. Though this looked like a dire prognosis, the reality
wasn’t as grim; in fact, it was scientifically enlightening. In a usual
brain with two hemispheres, visual stimuli from fibers of the right half of
the retina are funneled to the right hemisphere. Because Alice was missing
the right half of her brain, it actually learned to welcome the information
from the fibers of both the left and right parts of the retina into the
left hemisphere. This was an astonishing feat of brain self-modification,
upon which the author bases his hypothesis: Changes in the brain are driven
by neurons’ desire for space.
In your brain, neurons
aren’t speaking to each other in a cordial and cooperative manner—rather,
those neurons are negotiating, fiercely and violently for neuronal
territory. Neurobiologists David Hubel and Torsten Wiesel were the first to
demonstrate this aspect of the brain, gaining a Nobel Prize in 1981 for
their study of mammalian eyes. Another insight, this one by Rita
Levi-Montalcini, garnered yet another Nobel Prize in 1986. Levi-Montalcini
discovered what are now known as neurotrophins, the protein trophies that
give neurons life and inspire competition. Neurotrophins give neurons a
reason to fight—they’re the lifeblood of neuronal activity, and without
them, neurons perish.
Additionally, the author
notes that within the brain, neurons fall into one of two categories:
excitatory and inhibitory. As the names suggest, excitatory neurons get
their neighbors all riled up when they send messages to others, while
inhibitory neurons simply shut others down. The “livewired” brain is caught
in this fluctuating pendulum. Inhibitory neurons lay down deep routes,
dissuading otherwise possible connections from occurring. For instance,
Alice’s brain had the capability to reshape itself into a less conventional
form, but this was only realized when the quieter connections in her brain
were given a chance to perform, subsequently breeding axons for different
connections. In a usual brain, those secret routes are overrun by the
strength of other neurons. Rivalry both inhibits and instigates changes
within the brain.
For Alice, previous neural
tension actually fostered peace, proving that just like the rest of us,
neurons need their space.
|
|
6. Plasticity
lasts even into old age. All it takes is trying something new.
As we age, our neural
circuits fall into usual routines. Just as we wear the same brands of
clothes, take the same walks around our neighborhoods, and talk to the same
close friends, our neurons harden into place as we age. Our brains are
wired for this—using gathered information, they develop accurate
predictions in order to preserve energy. Novelty usually isn’t in the
picture. Though our brains do harden with time, the author argues that
plasticity differs by location. One part of the brain may be more or less
welcoming to change, depending upon the information it processes. For
instance, if a particular kind of information is relatively predictable in
your world, such as language, the part of the brain responsible for its
plasticity will be more difficult to change. But that’s only half the
story.
There’s so much information
swirling within our world that it’s possible for a brain to maintain a high
degree of plasticity despite its age. If a brain sees change as necessary
and if it’s constantly exposed to foreign experiences and new ways of
thinking, then it can preserve its elastic youth. In a research experiment
called the Nun Study, hundreds of Catholic nuns agreed to partake in
cognitive testing and a brain scan upon death. Despite their age, the nuns
remained intellectually bright, attending social events and completing
their chores with no mental difficulties. Brain autopsies revealed that
though one-third of those nuns maintained the neural damage indicative of
Alzheimer’s disease, they didn’t display any hints of mental lapse. Their
brains were always at the ready, soaking in new experiences, frustrations,
and life—with constant activity, their ability prolonged. Even in spite of
itself, the brain prevails.
We may have a difficult
time learning Mandarin as we get older, but we can still meet new people,
travel to foreign places, or at least take a new route to work. Stimulating
our brains with the new enables lifelong plasticity. Our brains are
powerful organs steeped in a world of constantly growing information vying
to assimilate into our realities. There’s so much left to discover within
the dark space between our ears and the bright atmosphere of the world
around us. It’s time to get started: Pick up an interesting hobby or
replace an old habit with something new. It may just change your life—and
your brain, too.
|
|
No comments:
Post a Comment